
Larger volumes of blood can be difficult to collect from pediatric and geriatric populations, and anxiety and fear associated with blood draws and needles can delay or prevent necessary patient care.
As a result, microsampling is increasingly being explored as an alternative to traditional phlebotomy collection.
Microsampling is a relatively pain-free and simplified method for collecting small volumes, on the scale of microliters, of blood, urine, and saliva matrices (1). Clinically, microsampling in the form of dried blood spots (DBS) remains limited to newborn screening, although many studies have demonstrated its value for various clinical applications.
This article provides an overview of the necessary considerations for implementing microsampling within a clinical laboratory, with a focus on DBS. Alternative dried matrices and blood microsampling methods that have the potential to overcome the limitations of DBS DBS are also highlighted.
Dried Blood Spots
DBS are the most well-known microsampling technique in the field of laboratory medicine. This sampling approach has been predominantly utilized in newborn screening programs, with emerging applications in pharmacokinetics (2), toxicology (2,3), and infectious disease (4,5). Table 1 describes the advantages and challenges associated with DBS as well as various clinical applications.
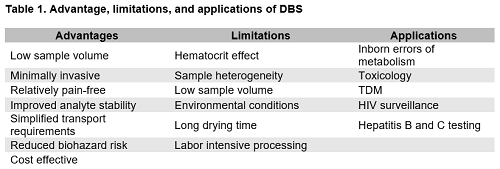
Collection Considerations
An average of 50−75 µL of blood is needed per DBS, with approximately 4−6 blood spots collected per patient for clinical testing (1,6). The collection process is fairly simple and relatively painless, involving a finger or heel stick followed by blotting the blood onto a collection card, typically Whatman 903 cards. A pipette or capillary tube can be used to transfer blood to the card. DBS can be collected without a trained phlebotomist or nurse and requires minimal materials compared wtih traditional phlebotomy collection, facilitating at-home self-collection of clinical specimens.
Several challenges are associated with DBS sampling that can affect downstream analytical measurements. The hematocrit effect is the most well-known disadvantage of DBS (1,4). “Milking” the puncture site, causes an increase in the percentage of interstitial fluid in the DBS and is the main cause of the hematocrit effect. Improper sample collection, age, and various clinical conditions can affect hematocrit, impact viscosity, and ultimately affect the spreadability of blood on the collection card. Sample heterogeneity also remains a challenge for DBS. Hematocrit, sample volume, and improper application of blood to the card can lead to a non-uniform distribution of analytes throughout the blood (1), and unlike conventional clinical specimens, mixing is not an option to achieve a homogenous sample matrix. Any sample outside the predefined target area will not be processed with the punched disc, affecting extraction recovery and analyte measurement. While these challenges represent a considerable barrier for widespread clinical use of DBS, emerging innovations and technological advancements are focused on mitigating these limitations (1).
Storage, Stability, and Transport
Following blood collection, the specimens are dried at room temperature for approximately 4 hours prior to shipment. Shipping and handling of DBS is simplified by the dried matrix, which inactivates most pathogens, reducing the biohazard risk typically associated with transport of clinical specimens (1,4). DBS also does not require refrigeration, dry ice, or overnight shipment to the testing laboratory and are considered non-regulated, exempt materials by the Centers for Disease Control and Prevention (CDC). As such, they can be shipped in ambient conditions in the mail or by other carriers (5). DBS sampling has become an area of increasing interest in global health and disease surveillance programs due to these technologically simple and cost-effective features (4,5).
While storage of DBS is conceptually simple, environmental field conditions during transport and in the laboratory as well as longer-term storage conditions can influence analyte measurement. High humidity and temperature can promote bacterial growth, impact drying rates, and alter analyte levels (1,4). Shipping guidelines provided by CDC describe ways to mitigate the impact of environmental conditions on analyte measurement, including the use of desiccants and humidity indicators to control and monitor moisture levels (1). However, a single set of storage conditions does not apply to all analytes. Extensive evaluation of analyte stability is therefore necessary to determine optimal storage conditions, transport requirements, and ensure accurate laboratory measurement. Beneficially, analytes susceptible to degradation via hydrolysis, esterase, or RNAase activity show improved stability in DBS compared with liquid specimens (1,6).
Sample Processing and Result Interpretation
Once in the testing laboratory, handling of DBS specimens is primarily manual, involving the removal of the DBS via a hole-punch device followed by analyte extraction, separation, and analytical measurement. Extraction typically includes incubation of the DBS punch with solvent, followed by mixing, centrifugation, and aspiration of the supernatant. However, the protocol will vary based on the analyte of interest, e.g., small molecules, RNA or DNA, or antigens/antibodies.
Manual DBS methods can be time and labor intensive, limiting the ability of labs to use DBS for high throughput clinical testing. Commercial systems for semiautomated and fully automated processing of DBS specimens are currently available and have been implemented into DBS workflows testing to increase throughput and minimize manual intervention (1). For example, the use of an automated puncher and liquid handling systems minimizes the number of manual processing steps, allowing for semiautomated workflows for DBS testing. Fully automated platforms streamline the clinical workflow even further, with the only manual step involving the insertion of the DBS cards into the system. Three commercially available systems, DBS-MS 500 (CAMAG), DBS Autosampler (Spark Holland), and the DSX-1 (Thermo Fisher) allow for fully automated extraction, separation, and analysis of DBS, although the specifications for each system differ. These three platforms are only compatible with mass spectrometry-based systems.
Proper result interpretation also represents a major barrier to widespread use of DBS within a clinical lab. Conventional clinical specimens include venous blood, plasma, or serum, while DBS use capillary blood. Inherent differences in sample type and composition as well as the drying effect can lead to differences in analyte concentration, making it difficult to compare DBS measurements to results from conventional clinical samples. Plasma equivalent results for DBS can assist in result interpretation, but the testing laboratory must generate and validate a conversion formula. This is not a new concept in laboratory medicine, as point-of-care glucose devices report plasma equivalent values for analytes measured in capillary blood. Alternatively, reflectance-based hematocrit measurements (7) and potassium levels (1) have been used to predict hematocrit in DBS, with the latter method providing a corrected analyte concentration for a hematocrit range of 0.19−0.63. Nevertheless, a comprehensive assessment of factors that may contribute to differences in DBS analyte levels due to sample matrix should be performed using matched DBS and plasma samples.
Current DBS Applications and Emerging Areas
Newborn Screening
Within a clinical lab, DBS is predominantly used for neonatal screening of inborn errors of metabolism. In the U.S., newborn screening programs may screen for over 60 diseases although the exact conditions vary from state to state (6). Each state provides specific guidelines on proper collection, storage, and transport of DBS for newborn screening. Conventionally, capillary blood from a heel stick is collected on a DBS collection card within 48 hours of birth and sent to the testing laboratory, typically a state laboratory. Newborn screening laboratories may have manual, semi-automated, or fully automated platforms to extract analytes from DBS. Measurement of analytes in DBS is most commonly performed using either flow injection or liquid chromatography tandem mass spectrometry (LC-MS/MS).
Infectious Disease
Given the key features of DBS sampling, there has been increasing interest in the use of DBS in global surveillance programs to monitor infectious diseases in resource-limited settings (4). In fact, the World Health Organization has recommended DBS for monitoring of hepatitis B and C and HIV to improve access to infectious disease screening in remote areas that lack clinical infrastructure (4,5).
Many studies have evaluated the performance of serological and nucleic acid tests for screening and confirmation of hepatitis using DBS. For screening of hepatitis B surface antigen in DBS, studies have reported pooled clinical sensitivities and specificities of 98% and 100%, respectively (5). Similar performance has been reported for hepatitis C antibodies (98% sensitivity and 99% specificity) (5). Researchers have evaluated the performance of various commercial HIV-1 RNA assays using DBS, too, with Abbott being the most used assay. Several studies have demonstrated that HIV RNA viral load can be reliably measured in DBS (4,5). However, extensive evaluation and validation of serology and nucleic acid testing of DBS in the testing laboratory under field conditions is necessary prior to implementation.
Toxicology and Therapeutic Drug Monitoring
DBS is an attractive sampling method for toxicology and therapeutic drug monitoring (TDM) applications. For toxicology, specifically, applications primarily include the assessment of substances of abuse, environmental contaminants, and trace metals in DBS (2). Numerous studies report the detection of various drugs of abuse in DBS, including cannabinoids, cocaine, opiates, amphetamine, and their metabolites (3). An advantage of using DBS for the detection of drugs of abuse compared with traditional whole blood specimens is improved stability of several drugs and metabolites, including cocaine and 6-monoacetylmorphine. Additionally, the dried matrix offers the benefit of minimizing enzyme activity, which is particularly useful in the measurement of phosphatidylethanol, a marker of alcohol consumption (3).
In addition to toxicology, there is growing interest in using DBS for TDM. DBS allow for convenient at-home sampling, requiring smaller volumes compared to venipuncture, which is highly desirable for TDM in pediatric patients. DBS-based TDM has been applied to various therapeutic drugs including antiretroviral, immunosuppressant, antimalarial, antibiotic, analgesic, and antidepressant drugs (2,3).
However, for both toxicology and TDM clinical use, a limitation of DBS is that they may require highly sensitive and selective instrumentation, such as LC-MS/MS, due to the small volume of sample provided on the collection cards.
Alternative Dried Matrices
Although DBS is the most widely used dried matrix in a clinical lab, dried urine spots (DUS) have shown promise for clinical applications and use. Within the last decade, dried saliva spots (DSS) have also been proposed as an alternative dried matrix. Both sampling methods offer the same benefits of DBS, such as simple transport and storage, reduced infection risk, and improved analyte stability, while allowing for noninvasive, pain-free, and more convenient sample collection.
The use of DUS for toxicology applications remains an active area of research as urine has a longer analyte detection window compared to blood and saliva (8). Several studies have shown the potential to detect various classes of drugs of abuse in DUS using LC-MS/MS (8). One study reported an 88% agreement for results from DUS and paired liquid urine specimens (9). Other studies have focused on the measurement of steroid hormones in DUS, with some proposing that collecting multiple DUS over a 24-hour period could serve as a surrogate for 24-hour urine specimens (10).
Recently, researchers have proposed using DSS for TDM, disease diagnosis, and cortisol measurement (11). This sample type has unique considerations compared to DBS and DUS. DSS has stricter patient preparation requirements; for example, patients should avoid tooth brushing and ingesting food and liquids for at least 30 minutes prior to specimen collection (11). The composition of the saliva, such as passive drool or stimulated saliva, can also affect analyte measurement (11). The sample volume of DSS ranges from 3-100 µL, depending on the detection method. Highly sensitive and selective instrumentation, such as tandem MS, may be necessary to detect analytes in smaller sample volumes given the generally lower analyte concentration in saliva compared to blood.
Blood Microsampling Alternatives
Within the last decade, multiple microsampling alternatives have been developed to address the major limitations associated with DBS while facilitating user-friendly and minimally invasive at-home specimen collection. These products include volumetric absorptive microsampling (VAMS), Tasso, and TAP blood collection devices (Figure 1). These products were developed to mitigate the limitations of DBS, such as hematocrit effect, volume, and heterogeneous analyte distribution.

VAMS
VAMS Mitra technology is manufactured by Neoteryx and uses a microsampling device consisting of a plastic handle and a polymeric tip to absorb a small volume of blood (10-30 µL) from a fingerstick. An advantage of VAMS over DBS is the elimination of the hematocrit effect; the porous Mitra tip absorbs a precise volume of blood independent of sample hematocrit (12). Similar to DBS, capillary blood is collected, and VAMS specimens must be dried at room temperature prior to storage and shipment. Once in the clinical lab, analytes are extracted from the Mitra tips via solvent extraction. For high throughput testing, analyte extraction can be expedited by placing VAMS specimens in Neoteryx 96-Autoracks, which are compatible with 96-channel pipettors and liquid handling systems. Neoteryx provides resources on different extraction methods for various analytes and assay types to guide use of VAMS in laboratory testing. VAMS has been used for TDM applications, showing promise for monitoring kinase inhibitors, immunosuppressants, antidepressants, antifungals, and antibiotics, among other analytes (12).
TAP and Tasso Devices
Yourbio Health and Tasso have recently developed microsampling devices that allow for at-home self-collection of whole blood liquid specimens. These devices are single use and operate in a similar multistep process: the upper arm is warmed to increase circulation, the device is held in place on the skin with adhesive, and a button is pressed to puncture the skin to collect the sample. While the collection process is more complex than DBS or VAMS technology, it is still relatively simple and pain-free. Compared to DBS, liquid blood specimens eliminate the additional steps needed to extract analytes from the collection cards, simplifying the processing workflow.
Yourbio Health currently has two devices available: the TAP, which the Food and Drug Administration (FDA) has cleared, and the TAP II device, which has only obtained the CE Mark. Both use a series of microneedles to puncture the skin. For the TAP device, up to 100 µL of capillary blood is collected in a reservoir that contains a lithium heparin chamber (12). The TAP II device enables collection of blood using a detachable tube—either an SST, red top, or lithium heparin tube, depending on the analyte of interest. Currently, Yourbio Health has TAP II kits available in the U.K. for at-home self-collection of blood (less than 250 µL) for SARS-CoV-2 antibody testing. The TAP device has recently been used for measurement of hemoglobin A1C (12).
Tasso manufactures three devices: the Tasso-SST, Tasso+, and Tasso-M20 (12). As the name implies, the Tasso-SST is comparable with a standard SST tube, allowing for the collection of 200-300 µL of blood within 5 minutes or less. Currently, this device is only available for investigational use only. With the Tasso+, serum, EDTA plasma, and heparinized plasma can be collected, expanding the number of analytes that can be measured using this technology. The Tasso+ can collect up to 600 µL of capillary blood and is currently FDA authorized. The Tasso-M20 enables the collection of four individual capillary DBS specimens within a single pod and is both FDA authorized and CE marked.
Recently, several studies have used the Tasso-SST device for anti-SARS-CoV-2 IgG antibody testing, with specimens collected in a self-administered, unsupervised manner (13). An overall accuracy of 98.9% was achieved for COVID-19 diagnosis compared with blood collected by standard venipuncture.
Conclusions
DBS has become increasingly popular within various clinical areas due to the method's at-home and remote self-collection capabilities, low volume requirements, and improved analyte stability, among other characteristics. Although there are several limitations associated with DBS, including hematocrit effect, volume, and heterogeneous analyte distribution, they are not insurmountable. Current technological advancements are focused on improving DBS as a sampling method to increase its utilization within multiple areas of the clinical lab.
Alternative blood microsampling techniques—namely, the Mitra, Tasso, and TAP devices—have also recently been developed to address the limitations of traditional DBS sampling. Outside of DBS, other dried matrices such as DUS and DSS are currently being evaluated for clinical applications. DUS and DSS offer the benefit of convenient, pain-free sample collection and are emerging as alternative dried specimens for clinical testing, although further validation is needed prior to clinical implementation
References
- Freeman JD, Rosman LM, Ratcliff JD, et al. State of the science in dried blood spots. Clin Chem 2018;64:656–79.
- Velghe S, de Troyer R, Stove C. Dried blood spots in therapeutic drug monitoring and toxicology. Expert Opin Drug Metab Toxicol 2018;14:1–3.
- Stove CP, Ingels ASME, de Kesel PMM, et al. Dried blood spots in toxicology: From the cradle to the grave? Crit Rev Toxicol 2012;42:230–43.
- Lim MD. Dried blood spots for global health diagnostics and surveillance: Opportunities and challenges. Am J Trop Med Hyg 2018;99:256–65.
- Tuaillon E, Kania D, Pisoni A, et al. Dried blood spot tests for the diagnosis and therapeutic monitoring of HIV and viral hepatitis B and C. Front Microbiol 2020;11:373.
- Chace DH, de Jesús VR, Spitzer AR. Clinical chemistry and dried blood spots: Increasing laboratory utilization by improved understanding of quantitative challenges. Bioanalysis 2014;6:2791–4.
- Capiau S, Wilk LS, Aalders MCG, et al. A novel, nondestructive, dried blood spot-based hematocrit prediction method using noncontact diffuse reflectance spectroscopy. Anal Chem 2016;88:6538–46.
- Tey HY, See HH. A review of recent advances in microsampling techniques of biological fluids for therapeutic drug monitoring. J Chromatogr A 2021;1635.
- Pablo A, Breaud AR, Clarke W. Automated analysis of dried urine spot (DUS) samples for toxicology screening. Clin Biochem 2020;75:70–7.
- Newman M, Curran DA, Mayfield BP. Dried urine and salivary profiling for complete assessment of cortisol and cortisol metabolites. J Clin Transl Endocrinol 2020;22.
- Han Y, Li X-L, Zhang M, et al. Potential use of a dried saliva
spot (DSS) in therapeutic
drug monitoring and disease diagnosis. J Pharm Anal 2021; doi:10.1016/j.jpha.2021.11.001.
- Delahaye L, Veenhof H, Koch BCP, et al. Alternative sampling devices to collect dried blood microsamples: State-of-the-art. Ther Drug Monit 2021;43:310-21.
- Hendelman T, Chaudhary A, LeClair AC, et al. Self-collection of capillary blood using Tasso-SST devices for anti-SARS-CoV-2 IgG antibody testing. PLoS ONE 2021;16.
Kyana Garza, PhD, is a clinical chemistry fellow at Johns Hopkins University School of Medicine in Baltimore, Maryland. +Email: [email protected]
William Clarke, PhD, MBA, DABCC, is the director of clinical toxicology and point-of-care testing at Johns Hopkins Hospital, as well as a professor of pathology and the Vice Chair of Pathology for Quality and Regulatory Affairs at Johns Hopkins University School of Medicine in Baltimore, Maryland.+Email: [email protected]